Degradation, water uptake, injectability and mechanical strength of injectable bone substitutes composed of silk fibroin and hydroxyapatite nanorods
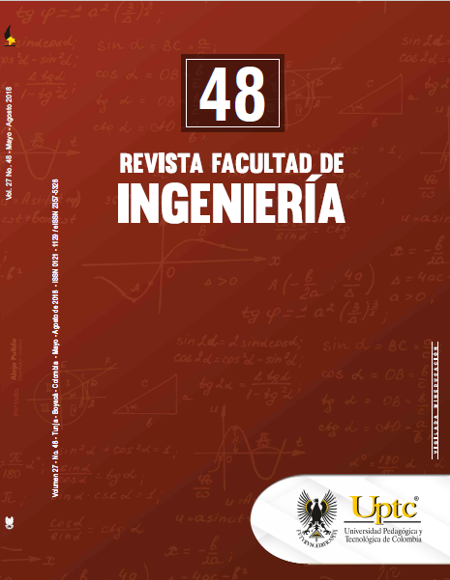
Abstract
Injectable bone substitutes are generally composite materials capable of being extruded through a device; they consist of a solid phase inside a matrix that allows the easy movement of particles. Injectable bone substitutes have the advantage of allowing its application in situ without the need of invasive surgical techniques, guaranteeing a good recovery; for this reason, they are a promising alternative to replace conventional techniques to repair bone defects. Conventional techniques include the use of allografts and autografts, which often cause adverse reactions, and are disadvantageous for both the patient and the doctor. Furthermore, there are no reports regarding bone substitute development in Colombia, creating the necessity to research composite materials that could become injectable bone substitutes. In this study, we manufactured injectable bone substitutes with hydroxyapatite and calcium phosphate, which is most similar to bone tissue, and synthesized them in nanorods with shape and size similar to the natural hydroxyapatite found inside the body. Additionally, we used extracted silk fibroin from silkworm cocoons of Bombyx mori, a natural polymer of protein nature with high mechanical properties and excellent biocompatibility. For the materials manufactured, we evaluated degradation, in a simulated body fluid (SBF) at normal body temperature, water uptake, injectability and mechanical strength. The manufactured bone substitutes showed good degradation and water uptake properties, an approximate 97% injectability, and low mechanical resistance, indicating promising properties to be used as an injectable bone substitute.
Keywords
biomaterials, bone substitutes, ceramics, composite materials, polymers
References
- B. F. Ricciardi, and M. P. Bostrom, “Bone graft substitutes: Claims and credibility,” Semin. Arthroplasty, vol. 24 (2), pp. 119–123, Jun. 2013. DOI: https://doi.org/10.1053/j.sart.2013.07.002. DOI: https://doi.org/10.1053/j.sart.2013.07.002
- A. Hokugo, T. Saito, A. Li, K. Sato, Y. Tabata, and R. Jarrahy, “Stimulation of bone regeneration following the controlled release of water-insoluble oxysterol from biodegradable hydrogel,” Biomaterials, vol. 35(21), pp. 5565–71, Jul. 2014. DOI: https://doi.org/10.1016/j.biomaterials.2014.03.018. DOI: https://doi.org/10.1016/j.biomaterials.2014.03.018
- K. Lee, et al., “Bone regeneration via novel macroporous CPC scaffolds in critical-sized cranial defects in rats,” Dent. Mater., vol. 30(7), pp. e199-207, Jul. 2014. DOI: https://doi.org/10.1016/j.dental.2014.03.008. DOI: https://doi.org/10.1016/j.dental.2014.03.008
- M. D’Este, and D. Eglin, “Hydrogels in calcium phosphate moldable and injectable bone substitutes: Sticky excipients or advanced 3-D carriers?,” Acta Biomater., vol. 9(3), pp. 5421–5430, Mar. 2013. DOI: https://doi.org/10.1016/j.actbio.2012.11.022. DOI: https://doi.org/10.1016/j.actbio.2012.11.022
- D. S. Morais, et al., “Development and characterization of novel alginate-based hydrogels as vehicles for bone substitutes.,” Carbohydr. Polym., vol. 95(1), pp. 134–42, Jun. 2013. DOI: https://doi.org/10.1016/j.carbpol.2013.02.067. DOI: https://doi.org/10.1016/j.carbpol.2013.02.067
- Z. Chen, et al., “Degradability of injectable calcium sulfate/mineralized collagen-based bone repair material and its effect on bone tissue regeneration,” Mater. Sci. Eng. C, vol. 45, pp. 94–102, Dec. 2014. DOI: https://doi.org/10.1016/j.msec.2014.08.060. DOI: https://doi.org/10.1016/j.msec.2014.08.060
- T. Kokubo (Ed.), Bioceramics and their clinical applications. Boston, USA: JMM, 2008. DOI: https://doi.org/10.1533/9781845694227
- J. Wasiak, A. K. Richmond, and B. Sc, “Bone Grafts and Bone Substitutes for Opening-Wedge Osteotomies of the Knee: A Systematic Review,” Arthrosc. J. Arthrosc. Relat. Surg., pp. 1–11, 2015.
- }
- R. A. Bhatt, and T. D. Rozental, “Bone Graft Substitutes,” Hand Clin., vol. 28(4), pp. 457–468, Nov. 2012. DOI: https://doi.org/10.1016/j.hcl.2012.08.001. DOI: https://doi.org/10.1016/j.hcl.2012.08.001
- S. Larsson, and G. Hannink, “Injectable bone-graft substitutes: Current products, their characteristics and indications, and new developments,” Injury, vol. 42(SUPPL. 2), pp. S30–S34, Sep. 2011. DOI: https://doi.org/10.1016/j.injury.2011.06.013. DOI: https://doi.org/10.1016/j.injury.2011.06.013
- I. M. Pelin, S. S. Maier, G. C. Chitanu, and V. Bulacovschi, “Preparation and characterization of a hydroxyapatite-collagen composite as component for injectable bone substitute,” Mater. Sci. Eng. C, vol. 29(7), pp. 2188–2194, Aug. 2009. DOI: https://doi.org/10.1016/j.msec.2009.04.021. DOI: https://doi.org/10.1016/j.msec.2009.04.021
- J. Melke, S. Midha, S. Ghosh, K. Ito, and S. Hofmann, “Silk fibroin as biomaterial for bone tissue engineering,” Acta Biomater., vol. 31, pp. 1–16, Feb. 2016. DOI: https://doi.org/10.1016/j.actbio.2015.09.005. DOI: https://doi.org/10.1016/j.actbio.2015.09.005
- M. Sadat-Shojai, M.-T. Khorasani, E. Dinpanah-Khoshdargi, and A. Jamshidi, “Synthesis methods for nanosized hydroxyapatite with diverse structures.,” Acta Biomater., vol. 9(8), pp. 7591–621, Aug. 2013. DOI: https://doi.org/10.1016/j.actbio.2013.04.012. DOI: https://doi.org/10.1016/j.actbio.2013.04.012
- B. Kundu, R. Rajkhowa, S. C. Kundu, and X. Wang, “Silk fibroin biomaterials for tissue regenerations,” Adv. Drug Deliv. Rev., vol. 65(4), pp. 457–470, Apr. 2013. DOI: https://doi.org/10.1016/j.addr.2012.09.043. DOI: https://doi.org/10.1016/j.addr.2012.09.043
- L.-D. Koh, et al., “Structures, Mechanical Properties and Applications of Silk Fibroin Materials,” Prog. Polym. Sci., pp. 1–25, 2015. DOI: https://doi.org/10.1016/j.progpolymsci.2015.02.001. DOI: https://doi.org/10.1016/j.progpolymsci.2015.02.001
- M. Buitrago, “Síntesis de nanofibras de fosfatos de calcio para su posible uso en sustitutos óseos," Thesis, Bioingeniera, Universidad de Antioquia, 2014.
- J. Hu, et al., “Injectable silk fibroin/polyurethane composite hydrogel for nucleus pulposus replacement,” J. Mater. Sci. Mater. Med., vol. 23(3), pp. 711–722, Mar. 2012. DOI: https://doi.org/10.1007/s10856-011-4533-y. DOI: https://doi.org/10.1007/s10856-011-4533-y
- P. Wang, B. Pi, J.-N. Wang, X.-S. Zhu, and H.-L. Yang, “Preparation and properties of calcium sulfate bone cement incorporated with silk fibroin and Sema3A-loaded chitosan microspheres,” Front. Mater. Sci., vol. 9(1), pp. 51–65, Mar. 2015. DOI: https://doi.org/10.1007/s11706-015-0278-8. DOI: https://doi.org/10.1007/s11706-015-0278-8
- M. A. de Moraes, G. M. Nogueira, R. F. Weska, and M. M. Beppu, “Preparation and characterization of insoluble silk fibroin/chitosan blend films,” Polymers (Basel)., vol. 2(4), pp. 719–727, Dec. 2010. DOI: https://doi.org/10.3390/polym2040719. DOI: https://doi.org/10.3390/polym2040719
- J. Jin, et al., “Transplantation of human placenta-derived mesenchymal stem cells in a silk fibroin/hydroxyapatite scaffold improves bone repair in rabbits,” J. Biosci. Bioeng., vol. 118(5), pp. 593–598, Nov. 2014. DOI: https://doi.org/10.1016/j.jbiosc.2014.05.001. DOI: https://doi.org/10.1016/j.jbiosc.2014.05.001
- M. Ribeiro et al., “Development of silk fibroin/nanohydroxyapatite composite hydrogels for bone tissue engineering,” Eur. Polym. J., vol. 67, pp. 66–77, Jun. 2015. DOI: https://doi.org/10.1016/j.eurpolymj.2015.03.056. DOI: https://doi.org/10.1016/j.eurpolymj.2015.03.056
- S. Ghanaati, et al., “An injectable bone substitute composed of beta-tricalcium phosphate granules, methylcellulose and hyaluronic acid inhibits connective tissue influx into its implantation bed in vivo,” Acta Biomater., vol. 7(11), pp. 4018–4028, Nov. 2011. DOI: https://doi.org/10.1016/j.actbio.2011.07.003. DOI: https://doi.org/10.1016/j.actbio.2011.07.003
- C. Cao, H. Li, J. Li, C. Liu, H. Yang, and B. Li, “Mechanical reinforcement of injectable calcium phosphate cement/silk fibroin (SF) composite by mineralized SF,” Ceram. Int., vol. 40(9), PART A, pp. 13987–13993, Nov. 2014. DOI: https://doi.org/10.1016/j.ceramint.2014.05.123. DOI: https://doi.org/10.1016/j.ceramint.2014.05.123
- J. Hu, X. Cai, S. Mo, L. Chen, X. Shen, and H. Tong, “Fabrication and characterization of chitosan-silk fibroin/hydroxyapatite composites via in situ precipitation for bone tissue engineering,” Chinese J. Polym. Sci., vol. 33(12), pp. 1661–1671, Dec. 2015. DOI: https://doi.org/10.1007/s10118-015-1710-3. DOI: https://doi.org/10.1007/s10118-015-1710-3
- L. Chen, J. Hu, J. Ran, X. Shen, and H. Tong, “Preparation and evaluation of collagen-silk fibroin/hydroxyapatite nanocomposites for bone tissue engineering,” Int. J. Biol. Macromol., vol. 65, pp. 1–7, Apr. 2014. DOI: https://doi.org/10.1016/j.ijbiomac.2014.01.003. DOI: https://doi.org/10.1016/j.ijbiomac.2014.01.003
- ASTM International, “Standard Practice for In-Vitro Environmental Conditioning of Polymer Matrix Composite Materials and Implant Devices.” 2008.
- T. Kokubo, and H. Takadama, “How useful is SBF in predicting in vivo bone bioactivity?,” Biomaterials, vol. 27(15), pp. 2907–2915, May. 2006. DOI: https://doi.org/10.1016/j.biomaterials.2006.01.017. DOI: https://doi.org/10.1016/j.biomaterials.2006.01.017
- R. Silva, et al., “Soft-matrices based on silk fibroin and alginate for tissue engineering,” Int. J. Biol. Macromol., vol. 93, pp. 1420–1431, Dec. 2016. DOI: https://doi.org/10.1016/j.ijbiomac.2016.04.045. DOI: https://doi.org/10.1016/j.ijbiomac.2016.04.045
- H. G. Oliveira Barud, et al., “Preparation and characterization of a bacterial cellulose/silk fibroin sponge scaffold for tissue regeneration,” Carbohydr. Polym., vol. 128, pp. 41–51, Sep. 2015. DOI: https://doi.org/10.1016/j.carbpol.2015.04.007. DOI: https://doi.org/10.1016/j.carbpol.2015.04.007
- H. H. K. Xu, M. D. Weir, E. F. Burguera, and A. M. Fraser, “Injectable and macroporous calcium phosphate cement scaffold,” Biomaterials, vol. 27(24), pp. 4279–4287, Aug. 2006. DOI: https://doi.org/10.1016/j.biomaterials.2006.03.001. DOI: https://doi.org/10.1016/j.biomaterials.2006.03.001
- E. F. Burguera, H. H. K. Xu, and L. Sun, “Injectable Calcium Phosphate Cement: Effects of Powder to Liquid Ratio and Needle Size,” vol. 84(2), pp. 493–502, 2009. DOI: https://doi.org/10.1002/jbm.b.30896
- J. Liu, J. Li, J. Ye, and F. He, “Setting behavior, mechanical property and biocompatibility of anti-washout wollastonite/calcium phosphate composite cement,” Ceram. Int., vol. 42(12), pp. 13670–13681, Sep. 2016. DOI: https://doi.org/10.1016/j.ceramint.2016.05.165. DOI: https://doi.org/10.1016/j.ceramint.2016.05.165
- ASTM International, Standard Test Method for Monotonic Compressive Strength of Advanced Ceramics at Ambient Temperature. 2014.
- M. Sadat-Shojai, M. T. Khorasani, and A. Jamshidi, “Hydrothermal processing of hydroxyapatite nanoparticles - A Taguchi experimental design approach,” J. Cryst. Growth, vol. 361(1), pp. 73–84, Dec. 2012. DOI: https://doi.org/10.1016/j.jcrysgro.2012.09.010. DOI: https://doi.org/10.1016/j.jcrysgro.2012.09.010
- X.-Y. Zhao, et al., “Hydrothermal synthesis of hydroxyapatite nanorods using pyridoxal-5′-phosphate as a phosphorus source,” Mater. Res. Bull., vol. 55, pp. 67–70, Jul. 2014. DOI: https://doi.org/10.1016/j.materresbull.2014.04.008. DOI: https://doi.org/10.1016/j.materresbull.2014.04.008
- S. K. Padmanabhan, A. Balakrishnan, M.-C. Chu, Y. J. Lee, T. N. Kim, and S.-J. Cho, “Sol–gel synthesis and characterization of hydroxyapatite nanorods,” Particuology, vol. 7(6), pp. 466–470, Dec. 2009. DOI: https://doi.org/10.1016/j.partic.2009.06.008. DOI: https://doi.org/10.1016/j.partic.2009.06.008
- Y. Liu, et al., “Long-term biodistribution in vivo and toxicity of radioactive/magnetic hydroxyapatite nanorods.,” Biomaterials, vol. 35(10), pp. 3348–55, Mar. 2014. DOI: https://doi.org/10.1016/j.biomaterials.2013.12.064. DOI: https://doi.org/10.1016/j.biomaterials.2013.12.064
- W. P. S. L. Wijesinghe, et al., “Facile synthesis of both needle-like and spherical hydroxyapatite nanoparticles: Effect of synthetic temperature and calcination on morphology, crystallite size and crystallinity,” Mater. Sci. Eng. C, vol. 42, pp. 83–90, Sep. 2014. DOI: https://doi.org/10.1016/j.msec.2014.05.032. DOI: https://doi.org/10.1016/j.msec.2014.05.032
- S. C. Medoza Ruiz, and E. Delgado Mejía, "Propuesta y evaluación de una síntesis rápida y selectiva de algunos fosfatos de calcio por el método ácido-base," Universidad Nacional de Colombia, 2005.
- ASTM International, "Standard Specification for Composition of Ceramic Hydroxyapatite for Surgical Implants," F1185-88. 2014.
- Y. Cheng, L. Koh, D. Li, B. Ji, M. Han, and Y. Zhang, “On the strength of β -sheet crystallites of Bombyx mori silk fibroin,” Interface, vol. 11, 2014. DOI: https://doi.org/10.1098/rsif.2014.0305
- S. Xiao, Z. Wang, H. Ma, H. Yang, and W. Xu, “Effective removal of dyes from aqueous solution using ultrafine silk fibroin powder,” Adv. Powder Technol., vol. 25(2), pp. 574–581, Mar. 2014. DOI: https://doi.org/10.1016/j.apt.2013.09.007. DOI: https://doi.org/10.1016/j.apt.2013.09.007
- A. Teimouri, M. Azadi, R. Emadi, J. Lari, and A. N. Chermahini, “Preparation, characterization, degradation and biocompatibility of different silk fibroin based composite scaffolds prepared by freeze-drying method for tissue engineering application,” Polym. Degrad. Stab., vol. 121, pp. 18–29, Nov. 2015. DOI: https://doi.org/10.1016/j.polymdegradstab.2015.08.004. DOI: https://doi.org/10.1016/j.polymdegradstab.2015.08.004
- D. M. Zeng, J. J. Pan, Q. Wang, X. F. Liu, H. Wang, and K. Q. Zhang, “Controlling silk fibroin microspheres via molecular weight distribution,” Mater. Sci. Eng. C, vol. 50, pp. 226–233, May. 2015. DOI: https://doi.org/10.1016/j.msec.2015.02.005. DOI: https://doi.org/10.1016/j.msec.2015.02.005
- Q. Liu, Z. Chen, H. Pan, and B. W. Darvell, “The effect of excess phosphate on the solubility of hydroxyapatite,” Ceram. Int., vol. 40(2), pp. 2751–2761, Mar. 2014. DOI: https://doi.org/10.1016/j.ceramint.2013.10.044. DOI: https://doi.org/10.1016/j.ceramint.2013.10.044
- M. Andiappan, et al., “Electrospun eri silk fibroin scaffold coated with hydroxyapatite for bone tissue engineering applications,” Prog. Biomater., vol. 2(1), p. 6, 2013. DOI: https://doi.org/10.1186/2194-0517-2-6. DOI: https://doi.org/10.1186/2194-0517-2-6
- Z. Hadisi, J. Nourmohammadi, and J. Mohammadi, “Composite of porous starch-silk fibroin nanofiber-calcium phosphate for bone regeneration,” Ceram. Int., vol. 41(9), pp. 10745–10754, Nov. 2015. DOI: https://doi.org/10.1016/j.ceramint.2015.05.010. DOI: https://doi.org/10.1016/j.ceramint.2015.05.010
- G. Daculsi, “History of Development and Use of the Bioceramics and Biocomposites,” in Handbook of bioceramics and biocomposites, I. V. Antoniac, Ed. Rumania: Springer, 2016, pp. 1–1386. DOI: https://doi.org/10.1007/978-3-319-09230-0_2-2
- U. J. Kim, J. Park, H. Joo Kim, M. Wada, and D. L. Kaplan, “Three-dimensional aqueous-derived biomaterial scaffolds from silk fibroin,” Biomaterials, vol. 26(15), pp. 2775–2785, May. 2005. DOI: https://doi.org/10.1016/j.biomaterials.2004.07.044. DOI: https://doi.org/10.1016/j.biomaterials.2004.07.044
- M. P. Ginebra, A. Rilliard, E. Fernández, C. Elvira, J. San Román, and J. A. Planell, “Mechanical and rheological improvement of a calcium phosphate cement by the addition of a polymeric drug,” J. Biomed. Mater. Res., vol. 57(1), pp. 113–118, Oct. 2001. DOI: https://doi.org/10.1002/1097-4636(200110)57:1<113::AID-JBM1149>3.0.CO;2-5. DOI: https://doi.org/10.1002/1097-4636(200110)57:1<113::AID-JBM1149>3.0.CO;2-5
- M. Nordin and V. H. Frankel, Basic Biomechanics of the Musculoeskeletal System, 3rd ed. Lippincott Williams and Wilkins, 2001.
- M. E. Navarro Toro, "Desarrollo y caracterización de materiales biodegradables para regeneración ósea," Doctoral Thesis , Universidad Politecnica de Catalunya, 2005.
- J. González Ocampo, "Evaluación de las propiedades de cuerpos porosos de hidroxiapatita, obtenidos por gel-casting y su infiltración en espumas poliméricas," Master Thesis, Universidad de Antioquia, 2013.
- J. Black, and G. Hastings, Handbook of Biomaterial Properties. Boston: Chapman & Hall, 1998. DOI: https://doi.org/10.1007/978-1-4615-5801-9. DOI: https://doi.org/10.1007/978-1-4615-5801-9
- G. Tripathi, and B. Basu, “A porous hydroxyapatite scaffold for bone tissue engineering: Physico-mechanical and biological evaluations,” Ceram. Int., vol. 38(1), pp. 341–349, Jan. 2012. DOI: https://doi.org/10.1016/j.ceramint.2011.07.012. DOI: https://doi.org/10.1016/j.ceramint.2011.07.012