Protection of tomato plants against Fusarium oxysporum f. sp. lycopersici induced by chitosan
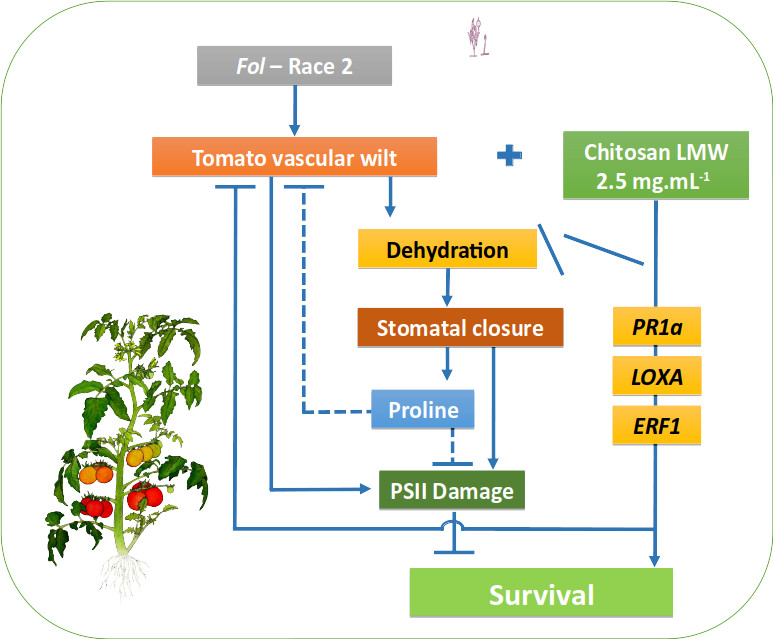
Abstract
Physiological processes of plants infected by vascular pathogens are mainly affected by vascular bundle obstruction, decreasing the absorption of water and nutrients and gas exchange by stomatal closure, and inducing oxidative cascades and PSII alterations. Chitosan, a derivative of chitin present in the cell wall of some organisms including fungi, induces plant defense responses, activating systemic resistance. In this study, three chitosan molecules (low, medium and high molecular weight) at different concentrations (0.5, 1, 1.5, 2, 2.5 and 3 mg mL-1) were assessed by in vitro tests against Fusarium oxysporum f. sp. lycopersici (Fol). Concentrations higher than 1 mg mL-1 were found to inhibit significantly the mycelial growth of Fol, with 95.8% of inhibition using chitosan with high molecular weight (3 mg mL-1). For in planta assays, chitosan treatment (low molecular weight 2.5 mg mL-1) showed significantly lower incidence and severity of wilting disease symptoms, 70 and 91%, respectively, compared to healthy plants used as a negative control. The effect of chitosan on the physiological and molecular responses of tomato plants infected with Fol was studied, evaluating the maximum potential quantum efficiency of PSII photochemistry (Fv/Fm), photochemical efficiency of PSII (Y(II)), stomatal conductance (gs), relative water content (RWC), proline content, photosynthetic pigments, dry mass, and differential gene expression (PAL, LOXA, ERF1, and PR1) of defense markers. A reduction of 70% in the incidence and 91% in the severity of the disease was achieved in plants treated with chitosan, mitigating the damage caused by Fol on Fv/Fm, Y(II), and chlorophyll contents by 23, 36, and 47%, respectively. Less impact was observed on gs, RWC, and dry mass (55, 11, and 26%, respectively). Chitosan-treated and Fol-infected plants over-expressed PR1a gene suggesting a priming-associated response. These results demonstrate the high potential of chitosan to protect tomato plants against Fol by regulating physiological and molecular responses in tomato plants.
Keywords
Vascular wilt, Resistance induction, Priming, Photosynthesis, Differential gene expression
References
Alsamir, M., T. Mahmood, N. Ahmad, and R. Trethowan. 2017. Distribution of organic metabolites after Fusarium wilt incidence in tomato (Solanum esculentum L.). Aust. J. Crop Sci. 11(9), 1123-1129. Doi: 10.21475/ajcs.17.11.09.pne536
Andersen, E., S. Ali, E. Byamukama, Y. Yen and M.P. Nepal. 2018. Disease resistance mechanisms in plants. Genes 9(7), 339. Doi: 10.3390/genes9070339
Ávila-Orozco, F.D., L.M. León-Gallón, M.I. Pinzón-Fandiño, A. Londoño-Orozco, and J.A. Gutiérrez-Cifuentes. 2017. Residualidad de fitosanitarios en tomate y uchuva cultivados en Quindío (Colombia). Cienc. Tecnol. Agropecu. 18(3), 571-582. Doi: 10.21930/rcta.vol18_num3_art:745
Bates, L.S., R.P. Waldren, and I.D. Teare. 1973. Rapid determination of free proline for water-stress studies. Plant Soil 39, 205-207. Doi: 10.1007/BF00018060
Carmona, S.L., D. Burbano-David, M.R. Gómez, W. Lopez, N. Ceballos, J. Castaño-Zapata, J. Simbaqueba, and M. Soto-Suárez. 2020. Characterization of pathogenic and nonpathogenic Fusarium oxysporum isolates associated with commercial tomato crops in the Andean region of Colombia. Pathogens 9,70. Doi: 10.3390/pathogens9010070
Chekali, S., S. Gargouri, T. Paulitz, J.M. Nicol, M. Rezgui, and B. Nasraoui. 2011. Effects of Fusarium culmorum and water stress on durum wheat in Tunisia. Crop Prot. 30(6), 718-725. Doi: 10.1016/j.cropro.2011.01.007
Chowdhury, S., A. Basu, and S. Kundu. 2017. Biotrophy-necrotrophy switch in pathogen evoke differential response in resistant and susceptible sesame involving multiple signaling pathways at different phases. Sci. Rep. 7, 17251. Doi: 10.1038/s41598-017-17248-7
Chun, S.C. and M. Chandrasekaran. 2019. Chitosan and chitosan nanoparticles induced expression of pathogenesis-related proteins genes enhances biotic stress tolerance in tomato. Int. J. Biol. Macromol. 125, 948-954. Doi: 10.1016/j.ijbiomac.2018.12.167
Beatrice, C., J.M.H. Linthorst, F. Cinzia, and R. Luca. 2017. Enhancement of PR1 and PR5 gene expressions by chitosan treatment in kiwifruit plants inoculated with Pseudomonas syringae pv. actinidiae. Eur. J. Plant Pathol. 147, 163-179. Doi: 10.1007/s10658-016-1080-x
Dean, R., J.A.L. Van Kan, Z.A. Pretorius, K.E. Hammond-Kosack, A. Di Pietro, P.D. Spanu, J.J. Rudd, M. Dickman, R. Kahmann, J. Ellis, and G.D. Foster. 2012. The top 10 fungal pathogens in molecular plant pathology. Mol. Plant Pathol. 13, 414-430. Doi: 10.1111/j.1364-3703.2011.00783.x
Duniway, J.M. 1971. Water relations of Fusarium wilt in tomato. Physiol. Plant Pathol. 1(4), 537-546. Doi: 10.1016/0048-4059(71)90015-4
Dzung, N.A., V.T.P. Khanh, and T.T. Dzung. 2011. Research on impact of chitosan oligomers on biophysical characteristics, growth, development and drought resistance of coffee. Carbohydr. Polym. 84(2), 751-755. Doi: 10.1016/j.carbpol.2010.07.066
El-Mohamedy, R.S.R., M.E.A. El-Aziz, and S. Kamel. 2019. Antifungal activity of chitosan nanoparticles against some plant pathogenic fungi in vitro. AgricEngInt: CIGR J. 21(4), 201-209.
Goltsev, V.N., H.M. Kalaji, M. Paunov, W. Bąba, T. Horaczek, J. Mojski, H. Kociel, and S.I. Allakhverdiev. 2016. Variable chlorophyll fluorescence and its use for assessing physiological condition of plant photosynthetic apparatus. Russ. J. Plant Physiol. 63, 869-893. Doi: 10.1134/S1021443716050058
Hernández-Lauzardo, A., S. Bautista-Baños, M.G. Velázquez-del Valle, M.G. Méndez-Montealvo, M.M. Sánchez-Rivera, L.A. Bello-Pérez. 2008. Antifungal effects of chitosan with different molecular weights on in vitro development of Rhizopus stolonifer (Ehrenb.: Fr.) Vuill. Carbohydr. Polym. 73(4), 541-547. Doi: 10.1016/j.carbpol.2007.12.020
Huang, Z., Z. Zhang, X. Zhang, H. Zhang, D. Huang, and R. Huang. 2004. Tomato TERF1 modulates ethylene response and enhances osmotic stress tolerance by activating expression of downstream genes. FEBS Lett. 573(1-3), 110-116. Doi: 10.1016/j.febslet.2004.07.064
Huot, B., J. Yao, B.L. Montgomery, and S.Y. He. 2014. Growth-defense tradeoffs in plants: A balancing act to optimize fitness. Mol. Plant 7(8), 1267-1287. Doi: 10.1093/mp/ssu049
Jamiołkowska, A. 2020. Natural compounds as elicitors of plant resistance against diseases and new biocontrol strategies. Agronomy 10(2), 173. Doi: 10.3390/agronomy10020173
Jelinski, N.A., K. Broz, W. Jonkers, L.J. Ma, and H.C. Kistler. 2017. Effector gene suites in some soil isolates of Fusarium oxysporum are not sufficient predictors of vascular wilt in tomato. Phytopathology 107(7), 842-851. Doi: 10.1094/PHYTO-12-16-0437-R
Jia, X., Q. Meng, H. Zeng, W. Wang, and H. Yin. 2016. Chitosan oligosaccharide induces resistance to Tobacco mosaic virus in Arabidopsis via the salicylic acid-mediated signalling pathway. Sci. Rep. 6, 26144. Doi: 10.1038/srep26144
Kappel, L., M. Münsterkötter, G. Sipos, C. Escobar Rodriguez, and S. Gruber. 2020. Chitin and chitosan remodeling defines vegetative development and Trichoderma biocontrol. PLoS Pathog. 16(2), e1008320. Doi: 10.1371/journal.ppat.1008320
Kardile, P.B, B.B. Dharbale, and M.V. Ugale. 2019. Chlorophyll fluorescence: a tool to study plant physiology and abiotic stress in plants. Indian J. Agric. Allied Sci. 5(2), 29-35.
Kauss, H., W. Jeblick, and A. Domard. 1989. The degrees of polymerization and N-acetylation of chitosan determine its ability to elicit callose formation in suspension cells and protoplasts of Catharanthus roseus. Planta 178, 385-392. Doi: 10.1007/BF00391866
Kumaraswamy, R.V., S. Kumari, R.C. Choudhary, A. Pal, R. Raliya, P. Biswas, and V. Saharan. 2018. Engineered chitosan based nanomaterials: Bioactivity, mechanisms and perspectives in plant protection and growth. Int. J. Biol. Macromol. 113, 494-506. Doi: 10.1016/j.ijbiomac.2018.02.130
León-Morcillo, R.J., J.A. Martín-Rodríguez, H. Vierheilig, J.A. Ocampo, and J.M. García-Garrido. 2012. Late activation of the 9-oxylipin pathway during arbuscular mycorrhiza formation in tomato and its regulation by jasmonate signalling. J. Exp. Bot. 63(10), 3545-3558. Doi: 10.1093/jxb/ers010
Lorenzini, G., L. Guidi, C. Nali, S. Ciompi, and G.F. Soldatini. 1997. Photosynthetic response of tomato plants to vascular wilt diseases. Plant Sci. 124(2), 143-152. Doi: 10.1016/S0168-9452(97)04600-1
Maluin, F.N. and M.Z. Hussein. 2020. Chitosan-based agronanochemicals as a sustainable alternative in crop protection. Molecules 25(7), 1611. Doi: 10.3390/molecules25071611
Martínez-Medina, A., I. Fernández, M.J. Sánchez-Guzmán, S.C. Jung, J.A. Pascual, and M.J. Pozo. 2013. Deciphering the hormonal signalling network behind the systemic resistance induced by Trichoderma harzianum in tomato. Front. Plant Sci. 4, 206. Doi: 10.3389/fpls.2013.00206
Maxwell, K. and G.N. Johnson. 2000. Chlorophyll a fluorescence - a practical guide. J. Exp. Bot. 51(345), 659-668. Doi: 10.1093/jxb/51.345.659
Melgarejo, L.M. (ed.). 2010. Experimentos en fisiología vegetal. Universidad Nacional de Colombia, Bogota.
Michielse, C.B. and M. Rep. 2009. Pathogen profile update: Fusarium oxysporum. Mol. Plant Pathol. 10, 311-324. Doi: 10.1111/j.1364-3703.2009.00538.x
Nogués, S., L. Cotxarrera, L. Alegre, and M.I. Trillas. 2002. Limitations to photosynthesis in tomato leaves induced by Fusarium wilt. New Phytol. 154(2), 461-470. Doi: 10.1046/j.1469-8137.2002.00379.x
Orzali, L., B. Corsi, C. Forni, and L. Riccioni. 2017. Chitosan in agriculture : A new challenge for managing plant disease. In: Shalaby, E. (ed.). Biological activities and application of marine polysaccharides. IntechOpen. Doi: 10.5772/66840
Pedroza-Sandoval, A. and J.A. Samaniego. 2009. Analisis del area bajo la curva del progreso de las enfermedades (ABCPE) en patosistemas agrícolas. pp.179-189. In: Bautista, N., L. Soto, and R. Pérez (eds.). Tópicos selectos de estadística aplicados a la fitosanidad. Colegio de postgraduados. Mexico, DF.
Pérez-Bueno, M.L., M. Pineda, and M. Barón. 2019. Phenotyping plant responses to biotic stress by chlorophyll fluorescence imaging. Front. Plant Sci. 10, 1135. Doi: 10.3389/fpls.2019.01135
Pshibytko, N.L., L.A. Zenevich, and L.F. Kabashnikova. 2006. Changes in the photosynthetic apparatus during Fusarium wilt of tomato. Russ. J. Plant Physiol. 53, 25-31. Doi: 10.1134/S1021443706010031
Rojas-Tapias, D., A. Moreno-Galván, S. Pardo-Díaz, M. Obando, D. Rivera, and R. Bonilla. 2012. Effect of inoculation with plant growth-promoting bacteria (PGPB) on amelioration of saline stress in maize (Zea mays). Appl. Soil Ecol. 61, 264-272. Doi: 10.1016/j.apsoil.2012.01.006
Rongai, D., P. Pulcini, B. Pesce, and F. Milano. 2017. Antifungal activity of pomegranate peel extract against Fusarium wilt of tomato. Eur. J. Plant Pathol. 147, 229-238. Doi: 10.1007/s10658-016-0994-7
Segarra, G., E. Casanova, M. Avilés, and I. Trillas. 2010. Trichoderma asperellum strain T34 controls fusarium wilt disease in tomato plants in soilless culture through competition for iron. Microb. Ecol. 59, 141-149. Doi: 10.1007/s00248-009-9545-5
Solanki, V.H., R.M. Patel, and K.G. Patel. 2015. Biochemical alteration in tomato varieties resistant and susceptible to Fusarium oxysporum f. sp. lycopersici. J. Cell Tissue Res. 15(2), 5029-5036.
Soliman, M.H. and R.S.R. El-Mohamedy. 2017. Induction of defense-related physiological and antioxidant enzyme response against powdery mildew disease in okra (Abelmoschus esculentus L.) plant by using chitosan and potassium salts. Mycobiology 45(4), 409-420. Doi: 10.5941/MYCO.2017.45.4.409
Soto-Suárez, M., P. Baldrich, D. Weigel, I. Rubio-Somoza, and B.S. Segundo. 2017. The Arabidopsis miR396 mediates pathogen-associated molecular pattern-triggered immune responses against fungal pathogens. Sci. Rep. 7, 44898. Doi: 10.1038/srep44898
Srinivas, C., D.N. Devi, K.N. Murthy, C.D. Mohan, T.R. Lakshmeesha, B.P. Singh, N.K. Kalagatur, S.R. Niranjana, A. Hashem, A.A. Alqarawi, B. Tabassum, E.F- Abd-Allah, S.C. Nayaka, and R.K. Srivastava. 2019. Fusarium oxysporum f. sp. lycopersici causal agent of vascular wilt disease of tomato: Biology to diversity – A review. Saudi J. Biol. Sci. 26(7), 1315-1324. Doi: 10.1016/j.sjbs.2019.06.002
Tikhonov, V.E., I.A. Yamskov, J. Palma-Guerrero, H.-B. Jansson, L.V. Lopez-Llorca, J. Salinas, D.V. Gerasimenko, I.D. Avdienko, and V.P. Varlamov. 2006. Bactericidal and antifungal activities of a low molecular weight chitosan and its N-/2(3)-(dodec-2-enyl) succinoyl/-derivatives. Carbohydr. Polym. 64(1), 66-72. Doi: 10.1016/j.carbpol.2005.10.021
Van, N.S., D.H. Minh, and N.D. Anh. 2013. Study on chitosan nanoparticles on biophysical characteristics and growth of Robusta coffee in green house. Biocatal. Agric. Biotechnol. 2(4), 289-294. Doi: 10.1016/J.BCAB.2013.06.001
Vargas-Hernandez, M., I. Macias-Bobadilla, R.G. Guevara-Gonzalez, S.J. Romero-Gomez, E. Rico-Garcia, R.V. Ocampo-Velazquez, L.L. Alvarez-Arquieta, and I. Torres-Pacheco. 2017. Plant hormesis management with biostimulants of biotic origin in agriculture. Front. Plant Sci. 8, 1762. Doi: 10.3389/fpls.2017.01762
Vidhyasekaran, P. 2016. Switching on plant innate immunity signaling systems - Bioengineering and molecular manipulation of PAMP-PIMP-PRR signaling complex. Springer, Cham, Switzerland. Doi 10.1007/978-3-319-26118-8
Wagner, A., W. Michalek, and A. Jamiolkowska. 2006. Chlorophyll fluorescence measurements as indicators of fusariosis severity in tomato plants. Agron. Res. 4(Special Issue), 461-464.
Walters, D., D. Walsh, A. Newton, and G. Lyon. 2005. Induced resistance for plant disease control: maximizing the efficacy of resistance elicitors. Phytopathology 95(12), 1368-1373. Doi: 10.1094/PHYTO-95-1368
Walters, D.R., J. Ratsep, and N.D. Havis. 2013. Controlling crop diseases using induced resistance: Challenges for the future. J. Exp. Bot. 64(5), 1263-1280. Doi: 10.1093/jxb/ert026
Wellburn, A.R. 1994. The spectral determination of chlorophylls a and b, as well as total carotenoids, using various solvents with spectrophotometers of different resolution. J. Plant Physiol. 144(3), 307-313. Doi: 10.1016/S0176-1617(11)81192-2
Xing, K., X. Zhu, X. Peng, and S. Qin. 2015. Chitosan antimicrobial and eliciting properties for pest control in agriculture: a review. Agron. Sustain. Dev. 35, 569-588. Doi: 10.1007/s13593-014-0252-3
Yadeta, K.A. and B.P.H. Thomma. 2013. The xylem as battleground for plant hosts and vascular wilt pathogens. Front. Plant Sci. 4, 97. Doi: 10.3389/fpls.2013.00097
Yockteng, R., A.M.R. Almeida, S. Yee, T. Andre, C. Hill, and C.D. Specht. 2013. A method for extracting high-quality RNA from diverse plants for next-generation sequencing and gene expression analyses. Appl. Plant Sci. 1(12), 1300070. Doi: 10.3732/apps.1300070
You, J., J. Zhang, M. Wu, L. Yang, W. Chen and, G. Li. 2016. Multiple criteria-based screening of Trichoderma isolates for biological control of Botrytis cinerea on tomato. Biol. Control 101, 31-38. Doi: 10.1016/j.biocontrol.2016.06.006
Zehra, A., M. Meena, and M.K. Dubey, M. Aamir, and R.S. Upadhyay. 2017. Synergistic effects of plant defense elicitors and Trichoderma harzianum on enhanced induction of antioxidant defense system in tomato against Fusarium wilt disease. Bot. Stud. 58, 44. Doi: 10.1186/s40529-017-0198-2
Zhang, A.X., P. Zou, and P. Li. 2017. Biostimulant activity of chitooligomers: Effect of different degrees of acetylation and polymerization on wheat seedlings under salt stress. Int. J. Agric. Biosyst. Eng. 4(12), 1.
Zhang, W., C. Qin, J. Zhao, and X. Wang. 2004. Phospholipase Dα1-derived phosphatidic acid interacts with ABI1 phosphatase 2C and regulates abscisic acid signaling. Proc. Natl. Acad. Sci. USA 101(25), 9508-9513. Doi: 10.1073/pnas.0402112101