Respuestas fisiológicas y bioquímicas del fríjol caupí (Vigna unguiculata L. Walp.) bajo déficit hídrico
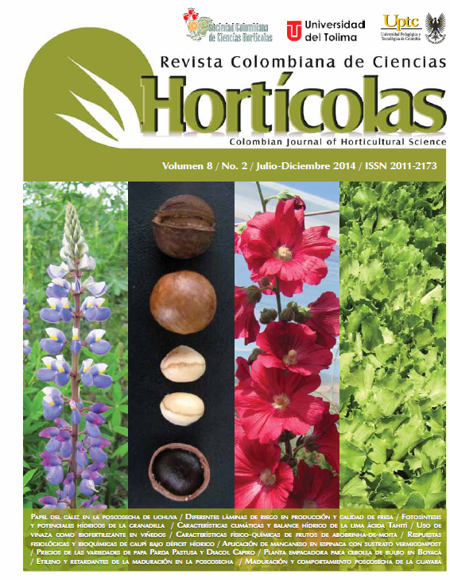
Resumen
El fríjol caupí contribuye a la alimentación humana y animal en muchas regiones del mundo, especialmente en aquellos lugares donde la sequía restringe la producción agrícola. El objetivo de este trabajo fue identificar algunas respuestas fisiológicas y bioquímicas de esta especie, bajo condiciones de estrés hídrico en fase reproductiva. Se experimentó bajo un diseño completamente aleatorizado en un arreglo factorial 2x6 (2 niveles de humedad del suelo y 6 genotipos) con tres repeticiones. Se analizó la respuesta de la especie evaluando el rendimiento de la planta mediante la cuantificación del rendimiento de grano/planta, el número de vainas/planta, el número de semillas/vaina, y la longitud de la vaina, se estimó la reducción relativa del rendimiento y la susceptibilidad a la sequía. También se analizó el intercambio gaseoso, la actividad de las enzimas catalasa y ascorbato peroxidasa, así como los contenidos de clorofila, carotenoides, proteínas libres, prolina y malondialdehído. El estrés por sequía causó una disminución en el rendimiento de grano/planta del 57,72%, número de vainas/planta del 49,40% y número de semillas/vaina del 32,07%. A los 17 días de sequía, la fotosíntesis empezó a registrar valores cercanos a cero cuando el contenido de humedad del suelo se redujo alrededor del 40% de la capacidad de campo,lo cual pudo ser ocasionado por limitaciones estomáticas y, posiblemente, por limitaciones no estomáticas. La proporción de clorofila a/clorofila b (Chla/Chlb), fue significativamente más baja, mientras que el contenido de malondialdehído fue significativamente más alto, en plantas bajo estrés hídrico.Palabras clave
fisiología de cultivos, estrés hídrico, intercambio gaseoso, enzimas antioxidantes, prolina
Citas
- Abayomi, Y.A. y T.O. Abidoye. 2009. Evaluation of cowpea genotypes for soil moisture stress tolerance under screen house conditions. Afr. J. Plant Sci. 3(10), 229-237.
- Afshar, R.M., H. Hadi y A. Pirzard. 2013. Effect of nano-iron on the yield and yield component of cowpea (Vigna unguiculata) under end season water deficit. Int. J. Agric. Res. Rev. 3(1), 27-34.
- Agbicodo, E.M., C.A. Fatokun, S. Muranaka, R.G.F Visser y C.G. Linden Van Der. 2009. Breeding drought tolerant cowpea: constraints, accomplishments, and future prospects. Euphytica 167, 353-370. Doi: 10.1007/s10681-009-9893-8
- Ahmed, F.E. y A.S.H. Suliman. 2010. Effect of water stress applied at different stages of growth on seed yield and water-use efficiency of cowpea. Agric. Biol. J. North America 1(4), 534-540.
- Bastos, E.A., S. Nascimento, E. Silva, F. Freire y R. Gomide. 2011. Identification of cowpea genotypes for drought tolerance. Rev. Ciênc. Agron. 42(1), 100-107. Doi: 10.1590/S1806-66902011000100013
- Bates, L.S. 1973. Rapid determination of free proline for water stress studies. Plant Soil 39, 205-207. Doi: 10.1007/BF00018060
- Belko, N., M. Zaman-Allah, N. Cisse, N.N. Diop, G. Zombre, J.D. Ehlers y V. Vadez. 2012. Lower soil moisture threshold for transpiration decline under water deficit correlates with lower canopy conductance and higher transpiration efficiency in drought-tolerant cowpea. Funct. Plant Biol. 39(4), 306-322. Doi: 10.1071/FP11282
- Beltrano, J., M.G. Ronco y M.C. Arango. 2006. Soil drying and rewatering applied at three grain developmental stages affect differentially growth and grain protein deposition in wheat (Triticum aestivum L.). Braz. J. Plant Physiol. 18(2), 341-350. Doi: 10.1590/S1677-04202006000200011
- Blum, A. 2009. Effective use of water (EUW) and not water-use efficiency (WUE) is the target of crop yield improvement under drought stress. Field Crop. Res. 112, 119-123. Doi: 10.1016/j.fcr.2009.03.009
- Bradford, M.M. 1976. A rapid and sensitive method for the quantitation of microgram quantities of protein utilizing the principle of protein-dye binding. Anal. Biochem. 72, 248-254. Doi: 10.1016/0003-2697(76)90527-3
- Brodribb, T. 1996. Dynamics of changing intercellular CO2 concentration (ci) during drought and determination of minimum functional ci. Plant Physiol. 111, 179-185.
- Cabrera, H.M. 2002. Respuestas ecofisiológicas de plantas en ecosistemas de zonas con clima mediterráneo y ambientes de alta montaña. Rev. Chil. Hist. Nat. 75, 625-637.
- Cakmak, I. y W.J. Horst. 1991. Effect of aluminium on lipid peroxidation, superoxide dismutase, catalase, and peroxidase activities in root tips of soybean (Glycine max). Physiol. Plant. 83, 463-468. Doi: 10.1111/j.1399-3054.1991.tb00121.x
- Cardona-Ayala, C.E., A.J. Jarma-Orozco, H. Araméndiz-Tatis, M. Perneth-Montaño y C.A. Vergara- Córdoba. 2013. Gas exchange and mass distribution of the cowpea (Vigna unguiculata -{L.}- Walp.) under water deficit. Agron. Colomb. 31(3), 288-296.
- Cardona-Ayala, C.E., A.J. Jarma-Orozco y H. Araméndiz-Tatis. 2013. Mecanismos de adaptación a sequía en fríjol caupí (Vigna unguiculata -{L.}- Walp.). Rev. Colomb. Cienc. Hortic. 7(2), 277-288.
- Claeys, H. y D. Inzé. 2013. The agony of choice: how plants balance growth and survival under water- limiting conditions. Plant Physiol. 162, 1768-1779. Doi: 10.1104/pp.113.220921
- Costa, R.C.L., A.K.S. Lobato, C.F. Oliveira Neto, P.SP. Maia, G.A.R Alves y H.D. Laughinghouse. 2008. Biochemical and physiological responses in two Vigna unguiculata (L.) Walp. cultivars under water stress. J. Agron. 7(1), 98-101. Doi: 10.3923/ja.2008.98.101
- Fernandes-Santos, C., G.P. Pereira Lima y L.B. Morgado. 2010. Tolerância e caracterização bioquímica em feijão-caupisubmetido a estressehídricona pré-floração. Naturalia 33, 34-44.
- Fisher, R.A. y R. Maurer. 1978. Drought resistance in spring wheat cultivars. Aust. J. Agric. Res. 29, 897-912. Doi: 10.1071/AR9791001
- Forsythe, W. 1985. Física de suelos: manual de labora- torio. IICA, San José, Costa Rica.
- Grassi, G. y F. Magnani. 2005. Stomatal, mesophyll conductance and biochemical limitations to photosynthesis as affected by drought and leaf ontogeny in ash and oak trees. Plant Cell Environ. 28, 834-849. Doi: 10.1111/j.1365-3040.2005.01333.x
- Havir, E.A. y N.A. McHale. 1987. Biochemical and developmental characterization of multiple forms of catalase in tobacco leaves. Plant Physiol. 84, 450-455. Doi: 10.1104/pp.84.2.450
- Ishiyaku, M.F. y H. Aliyu. 2013. Field evaluation of cowpea genotypes for drought tolerance and striga resistance in the dry savanna of the North-West Nigeria. Int. J. Plant Breed. Genet. 7(1), 47-56. Doi: 10.3923/ijpbg.2013.47.56
- Iturbe-Ormaetxe, I., P.R. Escuredo, C. Arrese-Igor y M. Becana. 1998. Oxidative damage in pea plants exposed to water deficit or paraquat. Plant Physiol. 116, 173-181. Doi: 10.1104/pp.116.1.173
- Lawlor, D.W. 2002. Limitation to photosynthesis in water-stressed leaves: stomata vs. metabolism and the role of ATP. Ann. Bot. 89, 871-885.
- Lichtenthaler, H.K. 1987. Chlorophylls and carotenoids: Pigments of photosynthetic biomembranes. Method. Enzymol. 148, 350-382.
- Martin, B. y N.A. Ruiz-Torres. 1992. Effects of water-deficit stress on photosynthesis, its components and component limitations, and on water use efficiency in wheat (Triticum aestivum L.). Plant Physiol. 100, 733-739. Doi: 10.1104/pp.100.2.733
- Nakano, Y. y K. Asada. 1981. Hydrogen peroxide is scavenged by ascorbate-specific peroxidase in spinach choloroplasts. Plant Cell Physiol. 22, 867-880.
- Pompelli, M., R. Barata; H. Vitorino, E. Gonçalves, E. Rolim, M. Santos, J. Almeida, V. Ferreira, E. Lemos y L. Endres. 2010. Photosynthesis, photoprotection and antioxidant activity of purging nut under drought deficit and recovery. Biomass Bioener. 34(8), 1207-1215. Doi: 10.1016/j.biombioe.2010.03.011
- Pungulani, L.L.M., J.P. Millner, W.M. Williams y M. Banda. 2013. Improvement of leaf wilting scoring in cowpea (Vigna sinensis (L.) Walp.): From qualitative scale to quantitative index. Aust. J. Crop Sci. 7(9), 1262-1269.
- Rosales-Serna, R., P. Ramírez-Vallejo, J.A. Acosta-Gallegos, F. Castillo-González y J.D. Kelly. 2000. Grain yield and drought tolerance of common bean under field conditions. Agrociencia 34(2), 153-165.
- Singh, S.K. y K.R. Reddy. 2011. Regulation of photosynthesis, fluorescence, stomatal conductance and water-use efficiency of cowpea (Vigna unguiculata (L.) Walp.) under drought. J. Photochem. Photobiol B: Biol.105, 40-50. Doi: 10.1016/j.jphotobiol.2011.07.001
- Taiz, L. y E. Zeiger. 2010. Plant physiology. 5th ed. Sinauer Associates, Redwood City, CA. pp. 591-623.
- Santos, C.F., G.P.P. Lima y L.B. Morgado. 2010. Tolerância e caracterização bioquímica em feijão-caupisubmetido a estresse hídrico na préfloração. Naturalia 33, 34-44.
- Smirnoff, N. 1993. The role of active oxygen in the response of plants to water deficit and desiccation (Tansley Review No. 52). New Phytol. 125, 27-58. Doi: 10.1111/j.1469-8137.1993.tb03863.x
- Tausz, M., H.S. Ircelj y D. Grill. 2004. The glutathione system as a stress marker in plant ecophysiology: is a stress-response concept valid? J. Exp. Bot. 55 (404), 1955-1962. Doi: 10.1093/jxb/erh194
- Tripathy, B.C. y R. Oelmüller. 2012. Reactive oxygen species generation and signaling in plants. Plant Signal. Behavior 7(12), 1621-1633. Doi: 10.4161/psb.22455
- Witta, S., L. Galiciab, J. Liseca, J. Cairnsc, A. Tiessend, J.L. Arause, N. Palacios-Rojasb y A.R. Fernie. 2012. Metabolic and phenotypic responses of greenhouse-grown maize hybrids to experimentally controlled drought stress. Mol. Plant 5(2), 401-417. Doi: 10.1093/mp/ssr102
Descargas
Los datos de descargas todavía no están disponibles.