Modulating the PGPR activity of Lysinibacillus pinottii sp. nov. PB211 through plant sensitivity/resistance to exogenous auxins
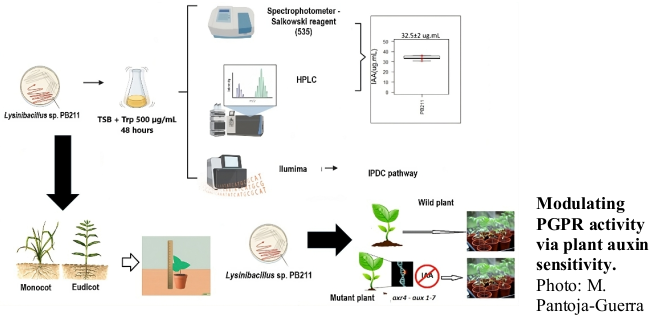
Abstract
The effectiveness of plant growth-promoting rhizobacteria (PGPR) strains indole-3-acetic acid (IAA) producers may be influenced by the plant's resistance to auxins. In this work, the impact of auxin resistance on the PGPR activity of Lysinibacillus pinottii sp. nov. PB211 was investigated. PB211 produced an average of 32 μg mL-1 of indole-3-acetic acid (IAA). Genetic evidence indicated that PB211 utilizes the indole pyruvic acid pathway for IAA synthesis. Regards to the response of plant models to IAA treatment, eudicot models (cucumber and bean) exhibited higher sensitivity to IAA compared to monocot models (corn and brachiaria). Monocots required higher IAA concentrations to elicit phenotypic changes in root architecture. The resistance/sensitivity of plants to exogenous auxins was found to modulate the PGPR activity of PB211. Inoculation with PB211 at varying concentrations resulted in differential effects on plant models. Eudicots displayed significant PGPR activity from lower inoculum concentrations, whereas monocots required higher inoculum concentrations to exhibit a similar consistent effect. The PB211 effect also was evaluated on Arabidopsis thaliana wild-type (col-0 “auxin-sensible”) and mutant (aux1-7/axr4-2 “auxin-resistant”) plants. PB211 had a “bell-shaped” effect on wild-type plants response, a typical response of auxin-activity, so, the PGPR effect decreased at the highest inoculum concentration. Conversely, the mutant plants exhibited increased PGPR activity with higher inoculum concentrations, compensating for their auxin-deficient phenotype. These findings suggest that the resistance/sensitivity of plants to exogenous auxins influences the effect of auxin-producer PGPRs. These relationships could facilitate the development and application of more effective biological inoculants for agriculture.
Keywords
Monocots, Eudicots, Indole-3-acetic acid, Plant growth promotion, Biofertilizers, Biostimulants
References
- Aloni, R. and T. Plotkin. 1985. Wound-induced and naturally occurring regenerative differentiation of xylem in Zea mays L. Planta 163(1), 126-132. Doi: https://doi.org/10.1007/bf00395906
- Alviar, K.B., K.M.R. Lum, J. Christine, and M.S. Pedro. 2021. Amplification and sequence analysis of indole-3-pyruvic acid (IPyA) pathway related genes from Bacillus spp. Biotechnology (Faisalabad) 20(1), 22-30. Doi: https://doi.org/10.3923/biotech.2021.22.30
- Balzan, S., G.S. Johal, and N. Carraro. 2014. The role of auxin transporters in monocots development. Front. Plant Sci. 5, 393. Doi: https://doi.org/10.3389/fpls.2014.00393
- Bashan, Y., L.E. De-Bashan, S.R. Prabhu, and J.-P. Hernandez. 2014. Advances in plant growth-promoting bacterial inoculant technology: formulations and practical perspectives (1998–2013). Plant Soil 378(1), 1-33. Doi: https://doi.org/10.1007/s11104-013-1956-x
- Bharucha, U., K. Patel, and U.B. Trivedi. 2013. Optimization of indole acetic acid production by Pseudomonas putida UB1 and its effect as plant growth-promoting rhizobacteria on mustard (Brassica nigra). Agric. Res. 2(3), 215-221. Doi: https://doi.org/10.1007/s40003-013-0065-7
- Blázquez, M.A., D.C. Nelson, and D. Weijers. 2020. Evolution of plant hormone response pathways. Annu. Rev. Plant Biol. 71(1), 327-353. Doi: https://doi.org/10.1146/annurev-arplant-050718-100309
- Blouin, M. 2018. Chemical communication: An evidence for co-evolution between plants and soil organisms. Appl. Soil Ecol. 123, 409-415. Doi: https://doi.org/10.1016/j.apsoil.2017.10.028
- Bunsangiam, S., N. Thongpae, S. Limtong, and N. Srisuk. 2021. Large scale production of indole-3-acetic acid and evaluation of the inhibitory effect of indole-3-acetic acid on weed growth. Sci. Rep. 11(1), 1-13. Doi: https://doi.org/10.1038/s41598-021-92305-w
- Calderon-Villalobos, L.I., X. Tan, N. Zheng, and M. Estelle. 2010. Auxin perception--structural insights. Cold Spring Harb. Perspect. Biol. 2(7), a005546. Doi: https://doi.org/10.1101/cshperspect.a005546
- Cao, J., G. Li, D. Qu, X. Li, and Y. Wang. 2020. Into the seed: Auxin controls seed development and grain yield. Int. J. Mol. Sci. 21(5), 1662. Doi: https://doi.org/10.3390/ijms21051662
- Castellano‐Hinojosa, A., V. Pérez‐Tapia, E.J. Bedmar, and N. Santillana. 2018. Purple corn-associated rhizobacteria with potential for plant growth promotion. J. Appl. Microbiol. 124(5), 1254-1264. Doi: https://doi.org/10.1111/jam.13708
- Chapman, E.J. and M. Estelle. 2009. Mechanism of auxin-regulated gene expression in plants. Annu. Rev. Genet. 43(1), 265-285. Doi: https://doi.org/10.1146/annurev-genet-102108-134148
- Chen, Y., Y. Xie, C. Song, L. Zheng, X. Rong, L. Jia, L. Luo, C. Zhang, X. Qu, and W. Xuan. 2018. A comparison of lateral root patterning among dicot and monocot plants. Plant Sci. 274, 201-211. Doi: https://doi.org/10.1016/j.plantsci.2018.05.018
- Conklin, P.A., J. Strable, S. Li, and M.J. Scanlon. 2019. On the mechanisms of development in monocot and eudicot leaves. New Phytol. 221(2), 706-724. Doi: https://doi.org/10.1111/nph.15371
- Dharmasiri, S., R. Swarup, K. Mockaitis, N. Dharmasiri, S.K. Singh, M. Kowalchyk, A. Marchant, S. Mills, G. Sandberg, M.J. Bennett, and M. Estelle. 2006. AXR4 is required for localization of the Auxin Influx Facilitator AUX1. Science 312(5777), 1218-1220. Doi: https://doi.org/10.1126/science.1122847
- Duca, D.R., D.R. Rose, and B.R. Glick. 2018. Indole acetic acid overproduction transformants of the rhizobacterium Pseudomonas sp. UW4. Antonie Van Leeuwenhoek 111(9), 1645-1660. Doi: https://doi.org/10.1007/s10482-018-1051-7
- Dunlap, C.A., E.T. Johnson, M. Burkett-Cadena, J. Cadena, and E.J. Muturi. 2024. Lysinibacillus pinottii sp. nov., a novel species with anti-mosquito and anti-mollusk activity. Antonie van Leeuwenhoek 117(1), 100. Doi: https://doi.org/10.1007/s10482-024-01993-7
- Fendrych, M., M. Akhmanova, J. Merrin, M. Glanc, S. Hagihara, K. Takahashi, N. Uchida, K.U. Torii, and J. Friml. 2018. Rapid and reversible root growth inhibition by TIR1 auxin signalling. Nat. Plants 4(7), 453-459. Doi: https://doi.org/10.1038/s41477-018-0190-1
- Glick, B.R., C. Liu, S. Ghosh, and E.B. Dumbroff. 1997. Early development of canola seedlings in the presence of the plant growth-promoting rhizobacterium Pseudomonas putida GR12-2. Soil Biol. Biochem. 29(8), 1233-1239. Doi: https://doi.org/10.1016/s0038-0717(97)00026-6
- Haga, K. and M. Lino. 1998. Auxin-growth relationships in maize coleoptiles and pea internodes and control by auxin of the tissue sensitivity to auxin. Plant Physiol. 117(4), 1473-1486. Doi: https://doi.org/10.1104/pp.117.4.1473
- Hobbie, L. and M. Estelle. 1995. The axr4 auxin-resistant mutants of Arabidopsis thaliana define a gene important for root gravitropism and lateral root initiation. Plant J. 7(2), 211-220. Doi: https://doi.org/10.1046/j.1365-313X.1995.7020211.x
- Jinal, H.N., K. Gopi, K. Kumar, and N. Amaresan. 2021. Effect of zinc-resistant Lysinibacillus species inoculation on growth, physiological properties, and zinc uptake in maize (Zea mays L.). Environ. Sci. Pollut. Res. 28(6), 6540-6548. Doi: https://doi.org/10.1007/s11356-020-10998-4
- Kaminsky, L.M., R.V. Trexler, R.J. Malik, K.L. Hockett, and T.H. Bell. 2019. The inherent conflicts in developing soil microbial inoculants. Trends Biotechnol. 37(2), 140-151. Doi: https://doi.org/10.1016/j.tibtech.2018.11.011
- Khan, A.L., B.A. Halo, A. Elyassi, S. Ali, K. Al-Hosni, J. Hussain, A. Al-Harrasi, and I.-J. Lee. 2016. Indole acetic acid and ACC deaminase from endophytic bacteria improves the growth of Solanum lycopersicum. Electron. J. Biotechnol. 21, 58-64. Doi: https://doi.org/10.1016/j.ejbt.2016.02.001
- Kim, W.-J. and H.-G. Song. 2012. Interactions between biosynthetic pathway and productivity of IAA in some rhizobacteria. Korean J. Microbiol. 48(1), 1-7. Doi: https://doi.org/10.7845/kjm.2012.48.1.001
- Kremer, R.J. 2007. Deleterious Rhizobacteria. pp. 335-357. In: Gnanamanickam, S.S. (ed.). Plant-associated bacteria. Springer, Dordrecht. Doi: https://doi.org/10.1007/978-1-4020-4538-7_10
- Kudoyarova, G., T. Arkhipova, T. Korshunova, M. Bakaeva, O. Loginov, and I.C. Dodd. 2019. Phytohormone mediation of interactions between plants and Non-Symbiotic growth promoting bacteria under edaphic stresses. Front. Plant Sci. 10, 1368. Doi: https://doi.org/10.3389/fpls.2019.01368
- Kudoyarova, G.R., L.B. Vysotskaya, T.N. Arkhipova, L.Yu. Kuzmina, N.F. Galimsyanova, L.V. Sidorova, I.M. Gabbasova, A.I. Melentiev, and S.Yu. Veselov. 2017. Effect of auxin producing and phosphate solubilizing bacteria on mobility of soil phosphorus, growth rate, and P acquisition by wheat plants. Acta Physiol. Plant. 39(11), 1-8. Doi: https://doi.org/10.1007/s11738-017-2556-9
- Kumar, M., V.P. Giri, S. Pandey, A. Gupta, M.K. Patel, A.B. Bajpai, S. Jenkins, and K.H.M. Siddique. 2021. Plant-Growth-Promoting rhizobacteria emerging as an effective bioinoculant to improve the growth, production, and stress tolerance of vegetable crops. Int. J. Mol. Sci. 22(22), 12245. Doi: https://doi.org/10.3390/ijms222212245
- Kunkel, B.N. and C.P. Harper. 2018. The roles of auxin during interactions between bacterial plant pathogens and their hosts. J. Exp. Bot. 69(2), 245-254. Doi: https://doi.org/10.1093/jxb/erx447
- Lavy, M. and M. Estelle. 2016. Mechanisms of auxin signaling. Development 143(18), 3226-3229. Doi: https://doi.org/10.1242/dev.131870
- Lee, R.D.-W. and H.-T. Cho. 2013. Auxin, the organizer of the hormonal/environmental signals for root hair growth. Front. Plant Sci. 4, 448. Doi: https://doi.org/10.3389/fpls.2013.00448
- Lobet, G., L. Pagès, and X. Draye. 2011. A novel image-analysis toolbox enabling quantitative analysis of root system architecture. Plant Physiol. 157(1), 29-39. Doi: https://doi.org/10.1104/pp.111.179895
- Lobet, G., M.P. Pound, J. Diener, C. Pradal, X. Draye, C. Godin, M. Javaux, D. Leitner, F. Meunier, P. Nacry, T.P. Pridmore, and A. Schnepf. 2015. Root system markup language: toward a unified root architecture description language. Plant Physiol. 167(3), 617-627. Doi: https://doi.org/10.1104/pp.114.253625
- Malik, D.K. and S.S. Sindhu. 2011. Production of indole acetic acid by Pseudomonas sp.: effect of coinoculation with Mesorhizobium sp. Cicer on nodulation and plant growth of chickpea (Cicer arietinum). Physiol. Mol. Biol. Plants 17(1), 25-32. Doi: https://doi.org/10.1007/s12298-010-0041-7
- Mangmang, J.S., R. Deaker and G. Rogers. 2015. Optimal plant growth-promoting concentration of Azospirillum brasilense inoculated to cucumber, lettuce and tomato seeds varies between bacterial strains. Isr. J. Plant Sci. 62(3), 145-152. Doi: https://doi.org/10.1080/07929978.2015.1039290
- Masuda, Y. 1980. Auxin-induced changes in noncellulosic polysaccharides of cell walls of monocot coleoptiles and dicot stems. pp. 79-89. In: Proc. 10th International Conference on Plant Growth Substances, Madison, WI. Doi: https://doi.org/10.1007/978-3-642-67720-5_7
- McSteen, P. 2010. Auxin and monocot development. Cold Spring Harb. Perspect. Biol. 2(3), a001479. Doi: https://doi.org/10.1101/cshperspect.a001479
- Mohite, B. 2013. Isolation and characterization of indole acetic acid (IAA) producing bacteria from rhizospheric soil and its effect on plant growth. J. Soil Sci. Plant Nutr. 13(3), 638-649. Doi: https://doi.org/10.4067/s0718-95162013005000051
- Moller, B. and D. Weijers. 2009. Auxin control of embryo patterning. Cold Spring Harb. Perspect. Biol. 1(5), a001545. Doi: https://doi.org/10.1101/cshperspect.a001545
- Naureen, Z., N.U. Rehman, H. Hussain, J. Hussain, S.A. Gilani, S.K.A. Housni, F. Mabood, A.L. Khan, S. Farooq, G. Abbas, and A.A. Harrasi. 2017. Exploring the potentials of Lysinibacillus sphaericus ZA9 for plant growth promotion and biocontrol activities against phytopathogenic fungi. Front. Microbiol. 8, 1477. Doi: https://doi.org/10.3389/fmicb.2017.01477
- Nehl, D.B., S.J. Allen, and J.F. Brown. 1997. Deleterious rhizosphere bacteria: an integrating perspective. Appl. Soil Ecol. 5(1), 1-20. Doi: https://doi.org/10.1016/S0929-1393(96)00124-2
- Nelissen, H., N. Gonzalez, and D. Inzé. 2016. Leaf growth in dicots and monocots: so different yet so alike. Curr. Opin. Plant Biol. 33, 72-76. Doi: https://doi.org/10.1016/j.pbi.2016.06.009
- Overvoorde, P., H. Fukaki, and T. Beeckman. 2010. Auxin control of root development. Cold Spring Harb. Perspect. Biol. 2(6), a001537. Doi: https://doi.org/10.1101/cshperspect.a001537
- Pal, A.K. and C. Sengupta. 2019. Isolation of cadmium and lead tolerant plant growth promoting rhizobacteria: Lysinibacillus varians and Pseudomonas putidafrom Indian agricultural soil. Soil Sediment Contam. Int. J. 28(7), 601-629. Doi: https://doi.org/10.1080/15320383.2019.1637398
- Pantoja-Guerra, M., M. Burkett-Cadena, J. Cadena, C.A. Dunlap, and C.A. Ramírez. 2023a. Lysinibacillus spp.: an IAA-producing endospore forming-bacteria that promotes plant growth. Antonie van Leeuwenhoek 116(7), 615-630. Doi: https://doi.org/10.1007/s10482-023-01828-x
- Pantoja-Guerra, M., N. Valero-Valero, and C.A. Ramírez. 2023b. Total auxin level in the soil–plant system as a modulating factor for the effectiveness of PGPR inocula: A review. Chem. Biol. Technol. Agric. 10(1), 6. Doi: http://doi.org/10.1186/s40538-022-00370-8
- Patten, C.L., A.J.C. Blakney, and T.J.D. Coulson. 2013. Activity, distribution and function of indole-3-acetic acid biosynthetic pathways in bacteria. Crit. Rev. Microbiol. 39(4), 395-415. Doi: https://doi.org/10.3109/1040841X.2012.716819
- Perrot-Rechenmann, C. 2010. Cellular responses to auxin: division versus expansion. Cold Spring Harb. Perspect. Biol. 2(5), a001446. Doi: https://doi.org/10.1101/cshperspect.a001446
- Phukan, J., J. Deka, K. Kurmi, and S. Kalita. 2021. Deleterious rhizobacteria as a potential bioherbicide-a review. Int. J. Agric. Environ. Sci. 8(2), 1-5. Doi: https://doi.org/10.14445/23942568/ijaes-v8i2p101
- Puga-Freitas, R., S. Barot, L. Taconnat, J.-P. Renou, and M. Blouin. 2012. Signal molecules mediate the impact of the earthworm Aporrectodea caliginosa on growth, development and defence of the plant Arabidopsis thaliana. PLoS One 7(12), e49504. Doi: https://doi.org/10.1371/journal.pone.0049504
- Ramírez, C.A. and J.W. Kloepper. 2010. Plant growth promotion by Bacillus amyloliquefaciens FZB45 depends on inoculum rate and P-related soil properties. Biol. Fertil. Soils 46(8), 835-844. Doi: https://doi.org/10.1007/s00374-010-0488-2
- Rivero, L., R. Scholl, N. Holomuzki, D. Crist, E. Grotewold, and J. Brkljacic. 2014. Handling Arabidopsis plants: growth, preservation of seeds, transformation, and genetic crosses. pp. 3-25. In: Sanchez-Serrano, J. and J. Salinas (eds.). Arabidopsis protocols. Methods in molecular biology. Vol. 1062. Humana Press, Totowa, NJ. Doi: https://doi.org/10.1007/978-1-62703-580-4_1
- Růzicka, K., K. Ljung, S. Vanneste, R. Podhorska, T. Beeckman, J. Friml, and E. Benkova. 2007. Ethylene regulates root growth through effects on auxin biosynthesis and transport-dependent auxin distribution. Plant Cell 19(7), 2197-2212. Doi: https://doi.org/10.1105/tpc.107.052126
- Scarpella, E. and A.H. Meijer. 2004. Pattern formation in the vascular system of monocot and dicot plant species. New Phytol. 164(2), 209-242. Doi: https://doi.org/10.1111/j.1469-8137.2004.01191.x
- Shao, J., S. Li, N. Zhang, X. Cui, X. Zhou, G. Zhang, Q. Shen, and R. Zhang. 2015. Analysis and cloning of the synthetic pathway of the phytohormone indole-3-acetic acid in the plant-beneficial Bacillus amyloliquefaciens SQR9. Microb. Cell Fact. 14(1), 130. Doi: https://doi.org/10.1186/s12934-015-0323-4
- Spaepen, S., S. Bossuyt, K. Engelen, K. Marchal, and J. Vanderleyden. 2014. Phenotypical and molecular responses of Arabidopsis thaliana roots as a result of inoculation with the auxin-producing bacterium Azospirillum brasilense. New Phytol. 201(3), 850-861. Doi: https://doi.org/10.1111/nph.12590
- Suarez, D.E.C., A. Gigon, R. Puga-Freitas, P. Lavelle, E. Velasquez, and M. Blouin. 2014. Combined effects of earthworms and IAA-producing rhizobacteria on plant growth and development. Appl. Soil Ecol. 80, 100-107. Doi: https://doi.org/10.1016/j.apsoil.2014.04.004
- Swarup, R., J. Kargul, A. Marchant, D. Zadik, A. Rahman, R. Mills, A. Yemm, S. May, L. Williams, P. Millner, S. Tsurumi, I. Moore, R. Napier, I.D. Kerr, and M.J. Bennett. 2004. Structure-function analysis of the presumptive Arabidopsis auxin permease AUX1. Plant Cell 16(11), 3069-3083. Doi: https://doi.org/10.1105/tpc.104.024737
- Tabassum, B., A. Khan, M. Tariq, M. Ramzan, M.S.I. Khan, N. Shahid, and K. Aaliya. 2017. Bottlenecks in commercialisation and future prospects of PGPR. Appl. Soil Ecol. 121, 102-117. Doi: https://doi.org/10.1016/j.apsoil.2017.09.030
- Vanneste, S. and J. Friml. 2009. Auxin: a trigger for change in plant development. Cell 136(6), 1005-1016. Doi: https://doi.org/10.1016/j.cell.2009.03.001
- Vernoux, T., F. Besnard, and J. Traas. 2010. Auxin at the shoot apical meristem. Cold Spring Harb. Perspect. Biol. 2(4), a001487. Doi: https://doi.org/10.1101/cshperspect.a001487
- Vissenberg, K., N. Claeijs, D. Balcerowicz, and S. Schoenaers. 2020. Hormonal regulation of root hair growth and responses to the environment in Arabidopsis. J. Exp. Bot. 71(8), 2412-2427. Doi: https://doi.org/10.1093/jxb/eraa048
- Woodward, A.W. 2005. AuxIn: Regulation, action, and interaction. Ann. Bot. 95(5), 707-735. Doi: https://doi.org/10.1093/aob/mci083
- Yue, J., X. Hu, and J. Huang. 2014. Origin of plant auxin biosynthesis. Trends Plant Sci. 19(12), 764-770. Doi: https://doi.org/10.1016/j.tplants.2014.07.004
- Zhang, P., T. Jin, S.K. Sahu, J. Xu, Q. Shi, H. Liu, and Y. Wang. 2019. The distribution of tryptophan-dependent indole-3-acetic acid synthesis pathways in bacteria unraveled by large-scale genomic analysis. Molecules 24(7), 1411. Doi: https://doi.org/10.3390/molecules24071411