Assessment of Burkholderia glumae control in rice (Oryza sativa) FEDEARROZ 67, using silver nanoparticles (AgNPs) under greenhouse conditions
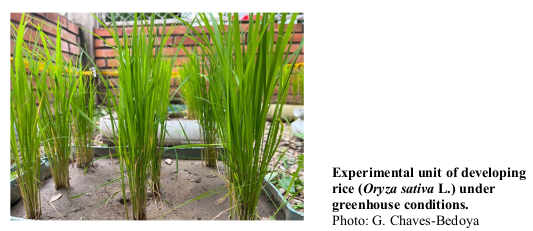
Abstract
Bacterial panicle blight, caused by Burkholderia glumae, represents a significant threat to global rice production, jeopardizing future food security. The severity of this seed-borne disease has been amplified by shifting environmental conditions, yet effective control strategies and fully-resistant rice varieties remain elusive. This research was conducted with the FEDEARROZ 67 rice variety sourced from Agua Clara, Cucuta municipality, and tests were undertaken under controlled greenhouse conditions at Universidad Francisco de Paula Santander. Our objective was to assess the potential of electrochemically synthesized silver nanoparticles (AgNPs) for controlling B. glumae. We employed a completely randomized design with five treatments: preventive, curative, positive control, negative control, and absolute control. Variables analyzed included the number of chlorotic leaves, plant height, and weight. Statistical analyses encompassed variance analysis and Tukey's mean comparison tests using XLSTATS version 2018. Our findings revealed that the preventive treatment with AgNPs at a 5 ppm concentration exhibited significant phytoprotective effects against B. glumae. Plants under this treatment showcased fewer chlorotic symptoms and greater heights and weights compared to controls. These findings underline the potential of nanotechnology as a groundbreaking tool for combating bacterial diseases in essential crops such as rice, thereby contributing to a sustainable future for global agriculture.
Keywords
Bacterial panicle blight, Rice disease, Bacterial control, Plant transformation
References
- Abeer Mohammed, A.B., M.M. Abd Elhamid, M.K.M. Khalil, A.S. Ali, and R.N. Abbas. 2022. The potential activity of biosynthesized silver nanoparticles of Pseudomonas aeruginosa as an antibacterial agent against multidrug-resistant isolates from intensive care unit and anticancer agent. Environ. Sci. Eur. 34(1), 109. Doi: https://doi.org/10.1186/s12302-022-00684-2
- Ahmed, T., Z. Wu, H. Jiang, J. Luo, M. Noman, M. Shahid, I. Manzoor, K.S. Allemailem, F. Alrumaihi, and B. Li. 2021. Bioinspired green synthesis of zinc oxide nanoparticles from a native Bacillus cereus strain RNT6: characterization and antibacterial activity against rice panicle blight pathogens Burkholderia glumae and B. gladioli. Nanomaterials 11(4), 884. Doi: https://doi.org/10.3390/nano11040884
- Almeida, A.S., A.C. Junior, and J.L. Bentes. 2021. Synthesis of silver nanoparticles (AgNPs) by Fusarium concolor and inhibition of plant pathogens. Summa Phytopathol. 47(1), 9-15. Doi: https://doi.org/10.1590/0100-5405/235097
- Anees Ahmad, S., S. Sachi Das, A. Khatoon, M. Tahir Ansari, M. Afzal, M.S. Hasnain, and A.K. Nayak. 2020. Bactericidal activity of silver nanoparticles: a mechanistic review. Materials Sci. Energy Technol. 3, 756-769. Doi: https://doi.org/https://doi.org/10.1016/j.mset.2020.09.002
- Chaves-Bedoya, G., H.A. Padilla-Sierra, L. Ortiz-Rojas, and G. Peña-Rodriguez. 2022. Potential use of electrochemically synthesized silver nanoparticles on rice panicle blight pathogen, Burkholderia glumae. Rev. Colomb. Cienc. Hortic. 16(3), e141738. Doi: https://doi.org/10.17584/rcch.2022v16i3.14738
- Dakal, T.C., A. Kumar, R.S. Majumdar, and V. Yadav. 2016. Mechanistic basis of antimicrobial actions of silver nanoparticles. Front. Microbiol. 7, 1831. Doi: https://doi.org/10.3389/fmicb.2016.01831
- Ferdous, Z. and A. Nemmar. 2020. Health impact of silver nanoparticles: a review of the biodistribution and toxicity following various routes of exposure. Int. J. Mol. Sci. 21(7), 2375. Doi: https://doi.org/10.3390/ijms21072375
- Flórez, N.M.V. and D. Uribe. 2011. Determinación de la infección de Burkholderia glumae en semillas de variedades comerciales colombianas de arroz. Rev. Fac. Nac. Agr. Medellin, 64(2), 6093-6104.
- Gupta, N., C.P. Upadhyaya, A. Singh, K.A. Abd-Elsalam, and R. Prasad. 2018. Applications of silver nanoparticles in plant protection. pp. 247-265. In: Abd-Elsalam, K.A. and R. Prasad (eds.). Nanobiotechnology applications in plant protection. Nanotechnology in the Life Sciences. Springer, Cham, Switzerland. Doi: https://doi.org/10.1007/978-3-319-91161-8_9
- He, H.-M., L.-N. Liu, S. Munir, N.H. Bashir, Y. Wang, J. Yang, and C.-Y. Li. 2019. Crop diversity and pest management in sustainable agriculture. J. Integ. Agric. 18(9), 1945-1952. Doi: https://doi.org/https://doi.org/10.1016/S2095-3119(19)62689-4
- Jacoby, G.A. 2005. Mechanisms of resistance to quinolones. Clin. Infect. Dis. 41(Suppl. 2), 120-126. Doi: https://doi.org/10.1086/428052
- Khan, M., A.U. Khan, N. Bogdanchikova, and D. Garibo. 2021. Antibacterial and antifungal studies of biosynthesized silver nanoparticles against plant parasitic nematode Meloidogyne incognita, plant pathogens Ralstonia solanacearum and Fusarium oxysporum. Molecules 26(9), 2462. Doi: https://doi.org/10.3390/molecules26092462
- Khan, S., M. Zahoor, R. Sher Khan, M. Ikram, and N.U. Islam. 2023. The impact of silver nanoparticles on the growth of plants: The agriculture applications. Heliyon 9(6), e16928. Doi: https://doi.org/10.1016/j.heliyon.2023.e16928
- Kim, S.W., J.H. Jung, K. Lamsal, Y.S. Kim, J.S. Min, and Y.S. Lee. 2012. Antifungal effects of silver nanoparticles (AgNPs) against various plant pathogenic fungi. Mycobiology 40(1), 53-58. Doi: https://doi.org/10.5941/myco.2012.40.1.053
- Mansoor, S., I. Zahoor, T.R. Baba, S.A. Padder, Z.A. Bhat, A.M. Koul, and L. Jiang. 2021. Fabrication of silver nanoparticles against fungal pathogens. Front. Nanotechnol. 3, 679358. Doi: https://doi.org/10.3389/fnano.2021.679358
- More, P.R., S. Pandit, A. De Filippis, G. Franci, I. Mijakovic, and M. Galdiero. 2023. Silver nanoparticles: bactericidal and mechanistic approach against drug resistant pathogens. Microorganisms 11(2), 369. Doi: https://doi.org/10.3390/microorganisms11020369
- Ortega, L. and C.M. Rojas. 2021. Bacterial panicle blight and Burkholderia glumae: from pathogen biology to disease control. Phytopathology 111(5), 772-778. Doi: https://doi.org/10.1094/PHYTO-09-20-0401-RVW
- Pedraza, L.A., J. Bautista, and D. Uribe-Vélez. 2018. Seed-born Burkholderia glumae infects rice seedling and maintains bacterial population during vegetative and reproductive growth stage. Plant Pathol. J. 34(5), 393-402. Doi: https://doi.org/10.5423/ppj.Oa.02.2018.0030
- Sayler, R.J., R.D. Cartwright, and Y. Yang. 2006. Genetic characterization and real-time PCR detection of Burkholderia glumae, a newly emerging bacterial pathogen of rice in the United States. Plant Dis. 90(5), 603-610. Doi: https://doi.org/10.1094/pd-90-0603
- Wang, L.L., C. Hu, and L.Q. Shao. 2017. The antimicrobial activity of nanoparticles: present situation and prospects for the future. Int. J. Nanomed. 12, 1227-1249. Doi: https://doi.org/10.2147/IJN.S121956
- Yan, A. and Z. Chen. 2019. Impacts of silver nanoparticles on plants: a focus on the phytotoxicity and underlying mechanism. Int. J. Mol. Sci. 20(5), 1003. Doi: https://doi.org/10.3390/ijms20051003
- Zhou, X.-G. 2019. Sustainable strategies for managing bacterial panicle blight in rice. In: Jia, Y. (ed.). Protecting rice grains in the post-genomic era. IntechOpen. Doi: https://doi.org/10.5772/intechopen.84882